"Advanced theoretical models and advanced simulation models for high-gradient superconducting future accelerators" – ATTAVANTI
Part of the cooperative project: "Technology of high-gradient superconducting future accelerators" – TOSCA
Prof. van Rienen's working group was able to acquire approx. 356,000 euros for its research in the field of accelerator physics and technology for the sub-project "Multiphysical shape optimisation of a quadrupole resonator under consideration of uncertainties" of the project "Innovative theoretical models and advanced simulation models for high-gradient superconducting future accelerators" (ATTAVANTI). The project is a part of the cooperative project "Technology of high-gradient superconducting future accelerators" (TOSCA), whose results are intended to increase the scientific performance of existing and planned large-scale accelerator facilities or those currently under construction. In the cooperative project TOSCA, the ATTAVANTI team led by Prof. Dr. Ursula van Rienen and co-project leader Jun.-Prof. Dr.-Ing. Simon Adrian is cooperating with partners from the TU Darmstadt, the University of Hamburg, the University of Mainz and the institutions CERN and DESY. In addition, web-based bi-weekly meetings are regularly organised by the partners at DESY to establish collaboration with scientists from HZB and Jefferson Lab (JLAB) from the USA.
The approved subproject improves the precision of measuring the surface resistance of superconducting materials by means of quadrupole resonators (QPRs). Thus, it is of general importance for current and future superconducting accelerators because QPRs enable the determination of many essential parameters of superconducting samples in a large parameter space. The obtained measurement results are crucial input parameters for design studies of various system components.
A postdoc is working on this research project at the University of Rostock and is in close contact with the collaborative partners.
In more detail:
QPRs are specialised devices used since the 1990s to measure the surface resistance of metal samples employing superconducting radio frequency (SRF) technology. The so-called calorimetric measurement allows an exact characterisation of flat metallic samples using QPRs. Figure 1 shows a schematic cross-section of the QPR setup developed by the HZB. Its structure consists of the following main components: the cavity, four quadrupole rods and the (calorimetric) sample chamber. Four vertical hollow rods made of niobium are collinearly arranged in the resonator. These rods are welded to the top plate of the QPR cavity. The lower end of the rods is bent into the semi-annular pole shoes and positioned at a low height above the sample's surface, which is attached to a replaceable cylinder. In the QPR, a quadrupole mode is used in the pole shoes, which in turn then causes a focused magnetic field in the specimen. The excited eddy currents lead to power dissipation and, thus, to the heating of the sample. The cavity and the calorimetric chamber with the material sample attached to the upper end are thermally insulated.
A heating regulator (PID) keeps the sample at a constant temperature from below. Finally, based on the "RF-DC compensation technique", the determined difference in the steady state of power consumption in RF and DC operation enables the precise (nΩ-resolution) determination of the surface resistance.
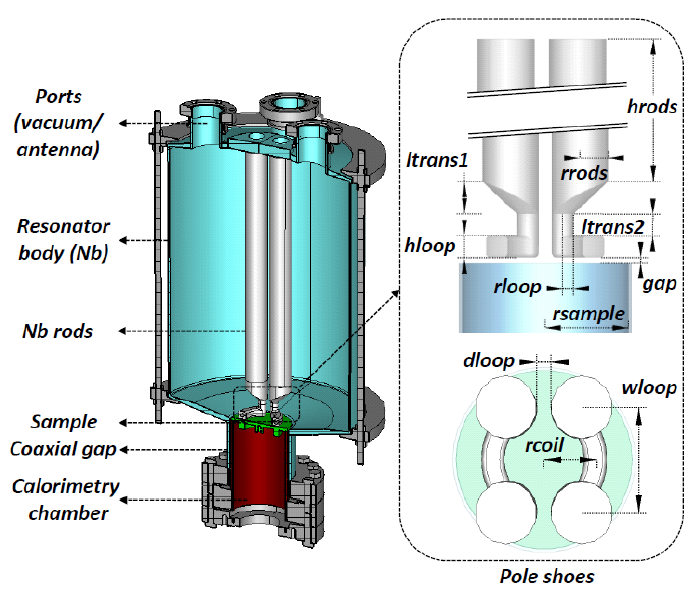
The field stability is a critical aspect of the QPR configuration shown in Figure 1. Specifically, it is sensitive to the Lorentz force and various external loads, causing significant surface resistance measurement errors, especially at the third harmonic frequency (1.3 GHz). A critical problem here is an unexpectedly high residual surface resistance at the third harmonic frequency, which seems to be associated with mechanical oscillations of the quadrupole rods in the order of 100 Hz. Such behaviour is noticeable in the QPRs used at CERN, even at all other operating frequencies.
In the design process of all QPRs so far, the focus has been exclusively on electromagnetic aspects, which means that ponderomotive effects and the Lorentz force detuning had not been taken into account.
The precise clarification of this issue and optimisation of the shape of the QPR based hereon are essential tasks that interest various accelerator laboratories - including CERN, HZB, DESY, and JLAB - with a view to the future. This gives rise to the task of modelling the multiphysical causes more precisely and thus being able to understand them better. This task requires thoroughly investigating the dynamic Lorentz force detuning and the microphonics effect in the time domain under electro-mechanical-thermal coupling to develop an efficient and robust E-M-T model in a steady state, including uncertainty of geometry and material parameters. Multiobjective shape optimisation is to be used to determine an optimal configuration that is insensitive to error-prone input parameters and allows compensation of the observed measurement distortion of the surface resistance at the third harmonic operating frequency.
Project duration: 01.07.2021 - 30.06.2024
Researcher: Dr. Piotr Putek
Project leadership: Prof. Dr. rer. nat. habil. Ursula van Rienen, Jun.-Prof. Dr.-Ing. Simon Adrian
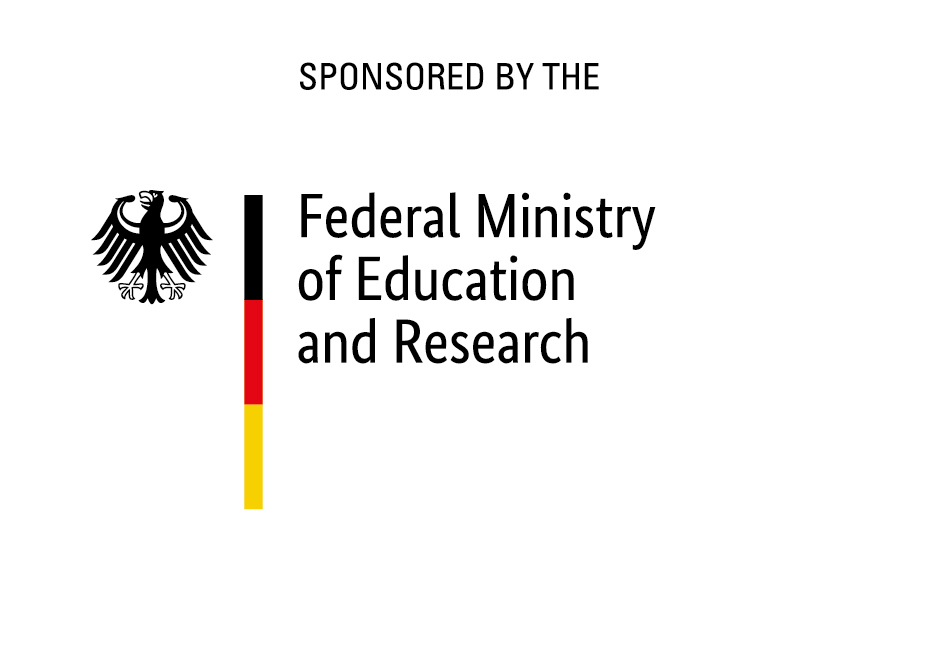